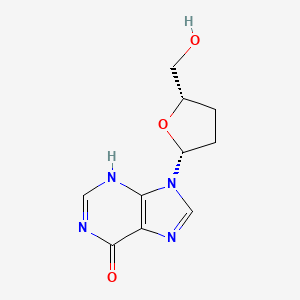
Didanosine
Overview
Description
Didanosine, also known as 2’,3’-dideoxyinosine, is a synthetic nucleoside analogue of adenosine. It is primarily used as an antiretroviral medication for the treatment of Human Immunodeficiency Virus (HIV) infection. This compound works by inhibiting the reverse transcriptase enzyme, which is essential for the replication of HIV. This compound was first described in 1975 and approved for use in the United States in 1991 .
Preparation Methods
Synthetic Routes and Reaction Conditions: Didanosine is synthesized through a multi-step process starting from inosine. The key steps involve the selective removal of hydroxyl groups at the 2’ and 3’ positions of the ribose moiety. This is typically achieved through a series of chemical reactions including protection, deprotection, and selective reduction steps.
Industrial Production Methods: Industrial production of this compound involves large-scale synthesis using similar chemical routes as in laboratory synthesis but optimized for higher yields and purity. The process includes rigorous quality control measures to ensure the final product meets pharmaceutical standards.
Types of Reactions:
Oxidation: this compound can undergo oxidation reactions, although these are not typically relevant to its pharmacological activity.
Reduction: The compound itself is a reduced form of inosine, achieved through selective reduction steps during synthesis.
Substitution: this compound can participate in nucleophilic substitution reactions, particularly at the purine ring.
Common Reagents and Conditions:
Oxidation: Common oxidizing agents like hydrogen peroxide or potassium permanganate.
Reduction: Reducing agents such as sodium borohydride or lithium aluminum hydride.
Substitution: Nucleophiles like amines or thiols under basic conditions.
Major Products Formed: The major product of these reactions is typically this compound itself or its derivatives, depending on the specific reaction conditions and reagents used.
Scientific Research Applications
Didanosine has a wide range of applications in scientific research:
Chemistry: Used as a model compound for studying nucleoside analogues and their chemical properties.
Biology: Employed in studies of nucleic acid metabolism and enzyme interactions.
Medicine: Extensively used in research on HIV treatment and resistance mechanisms.
Industry: Utilized in the development of antiretroviral therapies and in the study of drug-drug interactions.
Mechanism of Action
Didanosine is metabolized intracellularly to its active form, dideoxyadenosine triphosphate (ddATP). This active metabolite inhibits the HIV reverse transcriptase enzyme by competing with the natural substrate, deoxyadenosine triphosphate (dATP). By incorporating into the viral DNA, ddATP causes chain termination, preventing the virus from replicating .
Comparison with Similar Compounds
Zidovudine (AZT): Another nucleoside reverse transcriptase inhibitor used in HIV treatment.
Stavudine (d4T): Similar mechanism of action but different side effect profile.
Lamivudine (3TC): Often used in combination with other antiretrovirals for synergistic effects.
Uniqueness: Didanosine is unique in its specific structural modifications, which confer its activity against HIV. Unlike other nucleoside analogues, this compound has a hypoxanthine base attached to the sugar ring, which is crucial for its mechanism of action .
Biological Activity
Didanosine (ddI), a nucleoside reverse transcriptase inhibitor (NRTI), has been primarily utilized in the treatment of HIV/AIDS. Its biological activity is characterized by its mechanism of action, pharmacokinetics, mutagenicity, and clinical efficacy. This article synthesizes findings from various studies to provide a comprehensive overview of this compound's biological activity.
This compound is metabolized intracellularly to its active form, this compound triphosphate (ddATP). The conversion involves phosphorylation through several enzymatic pathways, ultimately leading to the incorporation of ddATP into viral DNA during reverse transcription. This incorporation results in chain termination, effectively inhibiting viral replication. The intracellular half-life of ddATP is approximately 12-24 hours, suggesting that less frequent dosing may be sufficient compared to other NRTIs like zidovudine (AZT) .
Pharmacokinetics
This compound exhibits low plasma protein binding (≤ 5%) and is less lipophilic than zidovudine. Consequently, it has a different distribution profile, with lower concentrations in cerebrospinal fluid compared to plasma . Approximately 40% of this compound is excreted unchanged in urine, while the remainder is metabolized into hypoxanthine and uric acid .
Mutagenicity and Toxicity
Research indicates that this compound may exhibit clastogenic effects at high doses. In vitro studies have shown it can induce DNA damage in human lymphocytes and Chinese hamster ovary cells. However, animal studies have reported no significant increase in micronuclei formation at lower doses . Notably, this compound does not appear to induce reverse mutations in Salmonella typhimurium without metabolic activation, suggesting a limited mutagenic potential under certain conditions .
Table 1: Summary of Mutagenicity Studies on this compound
Study Type | Results |
---|---|
In Vitro (Human Lymphocytes) | Clastogenic effects observed |
In Vitro (Chinese Hamster Ovary) | Induced DNA damage |
In Vivo (Rodent Micronucleus Assay) | No increase in micronuclei at low doses |
Salmonella typhimurium | No reverse mutations without activation |
Clinical Efficacy
This compound has demonstrated significant antiviral activity in clinical settings. A study involving 168 HIV-1 infected patients showed that those treated with this compound experienced a median decrease in HIV-1 RNA levels by -0.56 log copies/mL compared to +0.07 log copies/mL in the placebo group after four weeks . Notably, 31% of patients on this compound achieved undetectable viral loads (<400 copies/mL), compared to only 6% in the placebo group .
Case Study: this compound in Treatment-Experienced Patients
In a cohort of treatment-experienced patients with multiple nucleoside reverse-transcriptase inhibitor-associated mutations (NAMs), this compound maintained antiviral efficacy. Patients with up to five NAMs at baseline still exhibited significant reductions in viral load when treated with this compound . This underscores its potential utility even in challenging treatment scenarios.
Q & A
Basic Research Questions
Q. What validated analytical methods are recommended for quantifying didanosine in pharmaceutical formulations?
The United States Pharmacopeia (USP) outlines a reversed-phase HPLC method for this compound quantification. A standard preparation using USP this compound RS is diluted to ~LC/900, and the assay preparation follows similar dilution. Chromatographic separation uses a 0.01 M ammonium acetate buffer (pH 6.0) and a C18 column. Peak responses are compared between standard and test solutions to calculate purity (90–110% of labeled claim) . For higher sensitivity, LC-MS with deuterated internal standards (e.g., this compound-d2) improves accuracy in pharmacokinetic studies .
Q. How should researchers handle this compound to ensure safety in laboratory settings?
this compound requires precautions to avoid inhalation, skin contact, or ingestion. Use PPE (gloves, lab coat, goggles) and work in a ventilated hood. Storage should be in sealed containers at 15–30°C to prevent degradation. In case of exposure, rinse affected areas with water for 15 minutes and seek medical evaluation due to its acute toxicity (oral LD₅₀: 4300 mg/kg in rats) and potential mutagenicity .
Q. What are the key considerations when designing in vitro studies to assess this compound’s antiviral efficacy?
Use HIV-1 reverse transcriptase (RT) inhibition assays with wild-type and mutant RT strains (e.g., L74V or K65R mutations linked to resistance). Cell-based models (e.g., MT-4 lymphocytes) should measure IC₅₀ values via plaque reduction or RT activity assays. Include controls for cytotoxicity and validate results against reference inhibitors like zidovudine .
Q. How can researchers ensure stability of this compound in solution during long-term experiments?
Prepare fresh solutions in pH-neutral buffers (e.g., PBS) and avoid prolonged exposure to light or temperatures >30°C. Degradation products (e.g., hypoxanthine) can be monitored via HPLC with UV detection at 250 nm. For multi-day assays, freeze aliquots at -20°C and validate stability over 72 hours .
Advanced Research Questions
Q. How do RT codon polymorphisms (e.g., L228H/R) influence this compound’s virological response in clinical cohorts?
Retrospective genotypic analysis of HIV-1 isolates from ART-experienced patients reveals that polymorphisms at RT codons 228 (L→H/R) correlate with reduced response to this compound. Use multivariate regression to adjust for confounding factors (e.g., prior NRTI exposure). Annotate sequences with resistance scores (e.g., ANRS algorithm) and validate findings in independent datasets to account for population-specific mutation patterns .
Q. What methodologies resolve contradictions in long-term toxicity data for this compound in preclinical models?
Mitochondrial toxicity assays (e.g., lactate/pyruvate ratios in hepatocytes) and in vivo models (e.g., transgenic mice expressing POLG mutations) can clarify mechanisms like mitochondrial DNA depletion. Combine histopathology (e.g., hepatic steatosis) with metabolomics to distinguish drug-specific effects from confounding variables (e.g., comorbidities) .
Q. How can researchers optimize genotypic resistance scoring systems for this compound in heterogeneous HIV-1 subtypes?
Develop weighted scores using machine learning (e.g., random forests) trained on clinical outcomes (viral load reduction) and subtype-specific mutation frequencies. Cross-validate against global databases (e.g., Stanford HIVDB) and include accessory mutations (e.g., T69D) that modulate resistance. Ensure scores are updated as new subtype data emerge .
Q. What experimental approaches validate this compound’s degradation pathways under accelerated storage conditions?
Conduct forced degradation studies (heat, humidity, UV light) followed by LC-MS/MS to identify major degradants. Compare fragmentation patterns with reference standards (e.g., USP Related Compound A). Quantify degradation kinetics using Arrhenius modeling to predict shelf-life under standard storage .
Q. Methodological Best Practices
- Data Contradiction Analysis : Use meta-analytic frameworks (e.g., PRISMA) to harmonize disparate findings from clinical trials and preclinical studies. Stratify by variables like dosing regimen or patient CD4+ counts .
- Human Subject Research : Align participant selection with FDA guidelines (e.g., exclusion of HLA-B*5701-positive individuals to avoid hypersensitivity). Document informed consent and adverse events per IRB protocols .
Properties
IUPAC Name |
9-[5-(hydroxymethyl)oxolan-2-yl]-1H-purin-6-one | |
---|---|---|
Details | Computed by Lexichem TK 2.7.0 (PubChem release 2021.05.07) | |
Source | PubChem | |
URL | https://pubchem.ncbi.nlm.nih.gov | |
Description | Data deposited in or computed by PubChem | |
InChI |
InChI=1S/C10H12N4O3/c15-3-6-1-2-7(17-6)14-5-13-8-9(14)11-4-12-10(8)16/h4-7,15H,1-3H2,(H,11,12,16) | |
Details | Computed by InChI 1.0.6 (PubChem release 2021.05.07) | |
Source | PubChem | |
URL | https://pubchem.ncbi.nlm.nih.gov | |
Description | Data deposited in or computed by PubChem | |
InChI Key |
BXZVVICBKDXVGW-UHFFFAOYSA-N | |
Details | Computed by InChI 1.0.6 (PubChem release 2021.05.07) | |
Source | PubChem | |
URL | https://pubchem.ncbi.nlm.nih.gov | |
Description | Data deposited in or computed by PubChem | |
Canonical SMILES |
C1CC(OC1CO)N2C=NC3=C2N=CNC3=O | |
Details | Computed by OEChem 2.3.0 (PubChem release 2021.05.07) | |
Source | PubChem | |
URL | https://pubchem.ncbi.nlm.nih.gov | |
Description | Data deposited in or computed by PubChem | |
Molecular Formula |
C10H12N4O3 | |
Details | Computed by PubChem 2.1 (PubChem release 2021.05.07) | |
Source | PubChem | |
URL | https://pubchem.ncbi.nlm.nih.gov | |
Description | Data deposited in or computed by PubChem | |
DSSTOX Substance ID |
DTXSID80860902 | |
Record name | 9-[5-(Hydroxymethyl)oxolan-2-yl]-1,9-dihydro-6H-purin-6-one | |
Source | EPA DSSTox | |
URL | https://comptox.epa.gov/dashboard/DTXSID80860902 | |
Description | DSSTox provides a high quality public chemistry resource for supporting improved predictive toxicology. | |
Molecular Weight |
236.23 g/mol | |
Details | Computed by PubChem 2.1 (PubChem release 2021.05.07) | |
Source | PubChem | |
URL | https://pubchem.ncbi.nlm.nih.gov | |
Description | Data deposited in or computed by PubChem | |
CAS No. |
69655-05-6 | |
Record name | 2', hydrate | |
Source | DTP/NCI | |
URL | https://dtp.cancer.gov/dtpstandard/servlet/dwindex?searchtype=NSC&outputformat=html&searchlist=612049 | |
Description | The NCI Development Therapeutics Program (DTP) provides services and resources to the academic and private-sector research communities worldwide to facilitate the discovery and development of new cancer therapeutic agents. | |
Explanation | Unless otherwise indicated, all text within NCI products is free of copyright and may be reused without our permission. Credit the National Cancer Institute as the source. | |
Retrosynthesis Analysis
AI-Powered Synthesis Planning: Our tool employs the Template_relevance Pistachio, Template_relevance Bkms_metabolic, Template_relevance Pistachio_ringbreaker, Template_relevance Reaxys, Template_relevance Reaxys_biocatalysis model, leveraging a vast database of chemical reactions to predict feasible synthetic routes.
One-Step Synthesis Focus: Specifically designed for one-step synthesis, it provides concise and direct routes for your target compounds, streamlining the synthesis process.
Accurate Predictions: Utilizing the extensive PISTACHIO, BKMS_METABOLIC, PISTACHIO_RINGBREAKER, REAXYS, REAXYS_BIOCATALYSIS database, our tool offers high-accuracy predictions, reflecting the latest in chemical research and data.
Strategy Settings
Precursor scoring | Relevance Heuristic |
---|---|
Min. plausibility | 0.01 |
Model | Template_relevance |
Template Set | Pistachio/Bkms_metabolic/Pistachio_ringbreaker/Reaxys/Reaxys_biocatalysis |
Top-N result to add to graph | 6 |
Feasible Synthetic Routes
Disclaimer and Information on In-Vitro Research Products
Please be aware that all articles and product information presented on BenchChem are intended solely for informational purposes. The products available for purchase on BenchChem are specifically designed for in-vitro studies, which are conducted outside of living organisms. In-vitro studies, derived from the Latin term "in glass," involve experiments performed in controlled laboratory settings using cells or tissues. It is important to note that these products are not categorized as medicines or drugs, and they have not received approval from the FDA for the prevention, treatment, or cure of any medical condition, ailment, or disease. We must emphasize that any form of bodily introduction of these products into humans or animals is strictly prohibited by law. It is essential to adhere to these guidelines to ensure compliance with legal and ethical standards in research and experimentation.